BIOREACTORS IN MODERN MEDICINE – CONSIDERATION OF INTERRELATED FACTORS IN VIVO\IN VITRO
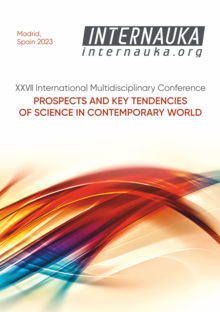
BIOREACTORS IN MODERN MEDICINE – CONSIDERATION OF INTERRELATED FACTORS IN VIVO\IN VITRO
Liza Dudaeva
Graduate student of National Research Nuclear University Moscow Engineering Physics Institute,
Russia, Moscow
The principles of early application of bioreactors were adapted for the purposes of tissue engineering, where the basic capabilities of bioreactors were clarified: the ability to evenly distribute cell mass, ensure the maintenance of cell viability factors (nutrients, oxygen, growth factors), increase mass transfer due to the mixing system of the culture medium. An important aspect is the control of ensuring reproducibility, including the effect of physical stimuli on the cell. [1]
Any bioreactor corresponds to the parameters of simplicity of design (to prevent contamination), a given device for a given range of applications, where the creation of optimal conditions includes oxygen saturation, power supply and removal of metabolites by evenly mixing the components of the contents of the bioreactor in the absence of thermal or mechanical action. action.
Taking into account the current trend towards in vitro research, the design of a bioreactor is most often carried out according to a specific model for a narrow range of applications, since in this case the best efficiency of work processes within the system most often complements each other without giving out a systematic error during use.
The complexity of the structure of human organs compensates[2] in vitro system – it allows you to standardize the internal model for a wide range of applications. In this case, the set of organs and tissues is separated from the adjacent biological space, taking into account the limitations [3,4,5] during testing for further interpretation of the results. The accuracy of reproducible experiments in the analysis of biological systems included in the system of living organisms directly affects scientific progress in assessing the safety of drugs and toxins, but such a system may not always be effective when prescribed to people with age[2] or characteristics other than experimental individuals. Therefore, under in vivo conditions, progress can be achieved up to a certain limit, or these conditions can only be an addition to the main experiment. The solution is to classify the medium [6,7] and sort it by in vitro parameters: a multiblock biological system contains the meaning of separating cells from the general balance, and then placing them through cultivation bowls into incubators for cultures for further maturation. The most important key to creating a realistic model in experimental scale conditions here is modeling the microenvironment of the cell. This allows you to create a stimulation similar to the human body for cellular growth.[8]
The type of stimuli is divided into three groups[9]: biochemical signals from other cells and the extracellular matrix, physical and structural stimuli from the 3D microenvironment, and flows of a physico-chemical pulse emanating from temperature gradients, concentrations [1,10].
Figure 1. Three axes of stimuli affecting body cells [1]
Stimuli act on cells, tissues, organs and organisms as time moves inexorably forward.
The tissue environment is distinguished by stimuli due to the cells themselves, the absorption of molecules during interaction with the extracellular environment is directly related to the scalable nature of the microenvironment. Often, the physical aspects also associated with the transfer flow[11] of molecules are of marginal importance, but in the three-dimensional scale of the experimental characteristics of the in vitro model can often be lost as unaccounted groups of negative influences (cell stimuli). However, it is in vitro models that contain the most accurate information thanks to such a set of simulated components [12,13,14,15] and are the most relevant in research.[16]
Figure 2. Application of in vitro models.[1]
The human body contains 50 trillion cells, if we consider that in the 3D version of the experiment, 1 cell consumes much lower oxygen than [17] in 2D, then the total metabolism of all cells is critically different in different variations of the models. The hierarchy of cells also adds differences in the parameters of a single functional unit, which allows us to introduce the concept of the smallest voxel of an autonomous organ, where cells grouped by functional and other characteristics of viability mimic the function of the organ. According to studies[2], in the absence of blood vessels, a voxel can be a cube with a side of about 200 microns or 8 × 10 -6 cm3 with the condition of cell survival in the absence of a blood grid, and taking into account the rule of "ten"[2] in 3D tissue studies[18], the minimum number of cells for cultivation is 56 000, where the cell density is min ~7 x 107 cells / cm3 (10% of the native cell plane), as a result, the minimum volume for in vitro models having a physiological value of 0.8 µl. Thus, we can equate to the minimum functional of the cell array for a successful in vitro experiment the figure of a parallelepiped with a size of 0.2x20x0.2 mm3. It is this volume and proportional to it that should be taken into account when designing a bioreactor for perfusing media[19].
It is important to take into account the separation of scaling, which is important when seeding cells through microchannels, for various functional experiments, critical concentration and pressure are sometimes used to overcome the maximum parameter of flow movement in the channels of microfluidic structures.[20] The above conditions applied to the system generalize the fact of their direct dependence on ensuring the necessary concentration of oxygen and nutrients, which should be taken into account when experimenting on an in vitro model in bioreactor devices.
References:
- Mattei G, Giusti S, Ahluwalia A. Design Criteria for Generating Physiologically Relevant In Vitro Models in Bioreactors. Processes. 2014; 2(3):548-569.
- Elliott, N.T.; Yuan, F. A review of three-dimensional in vitro tissue models for drug discovery and transport studies. J. Pharmcol. Sci. 2011, 100, 59–74.
- Bracken, M.B. Why animal studies are often poor predictors of human reactions to exposure. J. R. Soc. Med. 2009, 102, 120–122.
- Alberts, B.; Johnson, A.; Lewis, J.; Raff, M.; Roberts, K.; Walte, P. Molecular Biology of the Cell, 4th ed.; Garland Science: New York, NY, USA, 2002.
- Astashkina, A.; Mann, B.; Grainger, D.W. A critical evaluation of in vitro cell culture models for high-throughput drug screening and toxicity. Pharmacol. Ther. 2012, 134, 82–106.
- Nerem, R.M. Tissue engineering: The hope, the hype, and the future. Tissue Eng. 2006, 12, 1143–1150.
- Cimetta, E.; Godier-Furnémont, A.; Vunjak-Novakovic, G. Bioengineering heart tissue for in vitro testing. Curr. Opin. Biotechnol. 2013, 24, 926–932.
- Rouwkema, J.; Gibbs, S.; Lutolf, M.P.; Martin, I.; Vunjak-Novakovic, G.; Malda, J. In vitro platforms for tissue engineering: Implications for basic research and clinical translation. J. Tissue Eng. Regen. Med. 2011, 5, e164–e167.
- Di Nardo, P.; Minieri, M.; Ahluwalia, A. Engineering the Stem Cell Niche and the Differentiative Micro- and Macroenvironment: Technologies and Tools for Applying Biochemical, Physical and Structural Stimuli and Their Effects on Stem Cells. In Stem Cell Engineering; Springer: Heidelberg, Germany, 2011; pp. 41–59.
- Patzer, J.F. Oxygen consumption in a hollow fiber bioartificial liver. Artif. Organs 2004, 28, 83–98.
- Francioli, S.E.; Candrian, C.; Martin, K.; Heberer, M.; Martin, I.; Barbero, A. Effect of three-dimensional expansion and cell seeding density on the cartilage-forming capacity of human articular chondrocytes in type II collagen sponges. J. Biomed. Mater. Res. A 2010, 95, 924–931.
- Shoji, Y.; Nakashima, H. Nutraceutics and delivery systems. J. Drug Target. 2004, 12, 385–391.
- Seok, J.; Warren, H.S.; Cuenca, A.G.; Mindrinos, M.N.; Baker, H.V.; Xu, W.; Richards, D.R.; Gao, H.; Hennessy, L.; Finnerty, C.C.; et al. Genomic responses in mouse models poorly mimic human inflammatory diseases. Proc. Natl. Acad. Sci. USA 2013, 110, 3507–3512.
- Schyschka, L.; Sánchez, J.J.M.; Wang, Z.; Burkhardt, B.; Müller-Vieira, U.; Zeilinger, K.; Bachmann, A.; Nadalin, S.; Damm, G.; Nussler, A.K.; et al. Hepatic 3D cultures but not 2D cultures preserve specific transporter activity for acetaminophen-induced hepatotoxicity. Arch. Toxicol. 2013, 87, 1581–1593.
- Iwasa, J.; Ochi, M.; Uchio, Y.; Katsube, K.; Adachi, N.; Kawasaki, K. Effects of cell density on proliferation and matrix synthesis of chondrocytes embedded in atelocollagen gel. Artif. Organs 2003, 27, 249–255.
- Sung, J.H.; Shuler, M.L. In vitro microscale systems for systematic drug toxicity study. Bioprocess Biosyst. Eng. 2010, 33, 5–19.
- Sung, J.H.; Esch, M.B.; Shuler, M.L. Integration of in silico and in vitro platforms for pharmacokinetic-pharmacodynamic modeling. Expert Opin. Drug Metab. Toxicol. 2010, 6, 1063–1081.
- Loeve, S. About a Definition of Nano: How to Articulate Nano and Technology? Int. J. Philos. Chem. 2010, 6, 3–18.
- Lee, M.Y.; Kumar, R.A.; Sukumaran, S.M.; Hogg, M.G.; Clark, D.S.; Dordick, J.S. Three-dimensional cellular microarray for high-throughput toxicology assays. Proc. Natl. Acad. Sci. USA 2008, 105, 59–63.
- Sbrana, T.; Ahluwalia, A. Engineering Quasi-Vivo in vitro organ models. Adv. Exp. Med. Biol. 2012, 745, 138–153.