HYPOLIPIDEMIC AND ANTIDIABETIC EFFECTS OF METHANOLIC LEAF EXTRACT OF VERNONIA AMYGDALINA: STUDY ON ANIMAL MODEL
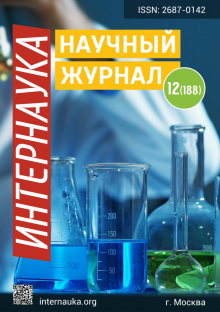
HYPOLIPIDEMIC AND ANTIDIABETIC EFFECTS OF METHANOLIC LEAF EXTRACT OF VERNONIA AMYGDALINA: STUDY ON ANIMAL MODEL
Pham Viet Cuong
Assoc. Prof., Ph.D, Mientrung Institute for Scientific Research, Vietnam Academy of Science and Technology, 321 Huynh Thuc Khang, Hue City,
Thua Thien Hue, Viet Nam
Tran Thi Hong
Ph.D, Mientrung Institute for Scientific Research, Vietnam Academy of Science and Technology, 321 Huynh Thuc Khang, Hue City,
Thua Thien Hue, Viet Nam
Trieu Ha Phuong
Bc., Institute of Biotechnology, Vietnam Academy of Science and Technology, 18 Hoang Quoc Viet, Nghia Do, Cau Giay, Hanoi, Viet Nam
Le Hoang Tuan Anh
Assoc. Prof., Ph.D, Mientrung Institute for Scientific Research, Vietnam Academy of Science and Technology, 321 Huynh Thuc Khang, Hue City,
Thua Thien Hue, Viet Nam
Do Thi Thao
Assoc. Prof., Ph.D, Institute of Biotechnology, Vietnam Academy of Science and Technology, 18 Hoang Quoc Viet, Nghia Do, Cau Giay,
Hanoi, Viet Nam
ABSTRACT
The leaves of Vernonia amygdalina are popularly used for variety of traditional medicinal purposes, of which is diabetic mellitus curing in Vietnam. Due to side effects of drug treatments or high carbohydrate and lipid consumed diet, these abnomal metabolic diseases has become more seriously, leading to the increased demand for the use of natural products to treat those of various ailments, including diabetes. This study was designed to evaluate the antidiabetic effects and hyperlipidemic reduction of methanolic leaf extract of V. amygdalina in vitro and on mice models. The obtained results showed that the leaf methanolic extract of V. amygdalina at the concentration of 500 µg/mL had inhibitory activities on both α-amylase and α-glucosidase. Besides, the extract at concentration of 400 mg kg-1 (body weight day-1) for 7 days, significantly reduced blood glucose level to 12.82 ± 1.10 mmol/L of induced hyperglycemic mice (P<0.05). The extract could also decreased total cholesterol, LDL-cholesterol and triglyceride levels in induced hyperlipidemic mice, compared with the pathological control at the same dose (P<0.05).
Keywords: diabetes, hyperglycemia, hypolipidemia, methanol extract, V. amygdalina.
I. INTRODUCTION
Type 2 diabetes is a condition in which cells can not use blood sugar (glucose) efficiently for energy and is characterized by high blood sugar, insulin resistance, and/or relative lack of insulin. It resulted in several serious consequences for brain, kidney, and cardiac (https://www.who.int/news). Together with the improved living condition over the world, the type 2 diabetic patients kept increasing each year [1]. Besides, the disease had a robust interaction with hyper-cholesterolaemia and liver failure. Diabetes was estimated as the most frequent origin of liver diseases and the secondary source of the presence of high blood cholesterol level that resulted to coronary artery problems [2,3]. Regarding to the type 2 diabetes, α-amylase and α-glucosidase were importain factors degrading polysacharide into glucose. The inhition of these two enzymes could decrease the blood glucose level in type 2 - diabetic patients. Currently, some medications have been used in clinical treatment for type 2 diabetes (for example: α-glucosidase inhibitor, biguanides, dopamine agonist…), but their grievous side effects were remained as anemia risk, neuropath risk, risk of liver disease, upset stomach, muscle weakness... [4,1].
Vernonia amygdalina is a shrub distributed mainly in tropical climates, a member of the Asteraceae family and a source of many local medicines in the Southeast Asia and West African sub-region for anti-diabetes, bacteria inhibition, anti-cancer, anti-obesity, anti-oxidant, liver protection… Several compounds isolated from the leaves of the Vietnamese Vernonia amygdalina Delile (Asteraceae) had shown promising α-amylase and α-glucosidase inhibition activity in-vitro, that played function in regulating plasma glucose [5,6,7]. In this paper, the methanol leaf extract from V. amygdalina Del. (VAM) was studied for its potential hyperlipidemic reduction and antihyperglycemic activities and synergistic action in oral glucose tolerance test in vivo.
II. MATERIALS AND METHODS
Preparation of leaf Extract: Fresh leaves of V. amygdalina were collected at Phu Vang Province, Thua Thien Hue, Central part of Vietnam and were identified by Dr. Nguyen The Cuong (Institute of Marine Biochemistry). The voucher number MISR-2017-14 was assigned.
Air-dried leaves of V. amygdalina (1.2 kg) were extracted with 100% methanol (5 L; 3 times) under sonication at 50oC for 4 h to yield a dark solid extract (200 g).
Chemicals and apparatus: Alloxan monohydrate (Sigma Aldrich) as agent of hyperglycemic inducer; Poloxamer 407 (P-407) as agent of hyperlipidemic inducer; NaCl 0.9%; KCl; Thiobarbituric acid (TBA), Tricloacetic acid (TCA) are all from Sigma Chemical Co. (St. Louis, MO., USA); Tris HCl buffer (Merk, Germany); Atorvastatin (tablets) 10 mg (Germany); were of high purity grade. OneTouch Ultra blood glucose meter; semi-automatic biochemical quantitative apparatus.
Animals:Young adult BALB/c mice (24±3 g) were supplied from Bioassay Laboratory, Institute of Biotechnology, Vietnam Academy Science and Technology. Animals were adapted to conditions of the controlled room temperature from 22oC to 25oC, 50-60% of humidity, 12 h day-night cycle. The animals were kept in large clean spacious cages and were given food and water ad libitum. The study was performed according to the guidelines of the Organization for Economic Cooperation and Development (OECD)(OECD, 2008) for the testing of chemicals, and the guidance for the care and use of laboratory animals published by the Animal Ethics Committee of Institute of Biotechnology, Vietnam Academy of Science and Technology
α-glucosidase inhibition: Glucosidase inhibition assay was carried out following the method of Elya et al. (2015) [8]. The methanol extract was dilluted with 0.1% dimethyl sulfoxide (DMSO) into different concentrations. Mixture of 50 µL of the sample with 100 μL of α-glucosidase (0.01 mg mL-1) in phosphate buffer (100 mM, pH 6.8) were incubated at 37oC for 10 min. Then, 50 μL of 5 mM substrate (p-nitrophenyl-α-D-glucopyranoside (pNPG)) were added to react with enzyme at 37oC for 60 min. After that, 50 μL of 0.1 M Na2CO3 was added to stop reaction. The optical density (OD) of the p-nitrophenol produced after the reaction was measured at 405 nm. The mean values were obtained from triplicate experiments. The enzyme inhibitory activity of the sample was evaluated as percentage inhibition using equation:
α-amylase inhibition: The methanol extract was dilluted with 0.1% dimethyl sulfoxide (DMSO) into several concentrations. 100 µL of the extract was added with 50 µL of amylase (0.5 mg mL-1) in 20 mM sodium phosphate buffer (pH 6.8) and 100 µL of 1% starch solution in 0.02 M sodium phosphate buffer (pH 6.9), then was incubated in 37oC for 15 minutes. The reaction was stopped with 250 µL of dinitrosalicyclic acid. The reaction mixture was then centrifuged to obtain the supernatant fluid which was measured at 540 nm. The mean of result were repeated three time of experiments. The control samples were prepared without any plant extracts/fractions/compounds. The percence of inhibition of amylase activity was calculated using the following formula:
Acute toxicity: Seventy BALB/c mice divided into 7 groups weighting 22-24 grams, undiscriminated male or fermale (n=10). Group 1 was served as biological control consuming 0.3 mL of distilled water. The other groups were treated in turn with 5; 6; 7; 8; 9; 10 g/kg (body weight (b.w.)) of VAM. The clinical signals and mortality were recorded and compared between samples and control during 7 days after treatment. The possible LD50 value was calculated using the Karber Behrens’ formula [9]: [LD50 = LD100 – Σ a×b/N] in which: LD50 is the dose killing half of the tested animals; LD100 is the lowest concentration killing all tested animals; N - the number of animals in a group; a and b are the differences between two consecutive doses and the mean mortality of two consecutive groups, respectively.
Effect on hyperglycemia: For hyperglycemia induction, a single dose of 150 mg/kg (b.w) of alloxan monohydrate was dissolved in physiological saline (0.9%) induced into the already fasted for 18 hours mice [10] by single intraperitoneal injection. One hour after injection, the mice were fed back with regular food. After 72 hours of injection, the blood glucose level was measured and the mice with blood glucose level higher than 150 mg L-1 were chosen for further study (WHO, 1985). Activity of MeOH extract on hypoglycemia was determined according Sivalingam and Sriram (2013) with minor modification [11]. Animals were divided into 5 groups (n=6) including the groups 1, 2 as negative and reference controls consuming water and 100 mg/kg (b.w day-1) Metformin, respectively. The groups 3, 4 and 5 were received VAM at the dose of 100 - 200 - 300 mg/kg b.w daily, respectively. After 7 days of VAM treatment, the weight and blood glucose levels of experimental animals were measured.
Regulating endogenous blood lipids: Poloxamer 407 (P-407) was used to induce endogenous hyperlipidemia in mice [12]. The study was performed according to the method of Seneni et al (2018) with some modifications [13]. The mice were divided into 04 experimental groups (n=6) in which group 1 and 2 (physiological and pathological controls) received distilled water, intra-peritoneal (i.p.) injection of saline and P-407 solution, respectively. Group 3 (reference group) was treated with Atorvastatin (50 mg kg-1 b.w.) by oral gavage and i.p. injected P-407 solution; group 4 was received VAM at 400 mg kg-1 b.w. by oral gavage and i.p. injected P-407 solution. P-407 was freshly prepared at 30% (w/v) concentration in cold water and injected i.p. at a single dose of 600 mg/kg. Mice were treated with VAM, Atorvastatin or distilled water after 2 hours of injection of P-407. After 24 hours of P-407 injection, total cholesterol, triglycerides, LDL-cholesterol and HDL-cholesterol in blood samples of all mice were measured.
Anti-glucose tolerance: Eighteen mice were divided into 3 groups (n=6). Already 18 hours fasted animals were treated with VAM at a concentration of 400 mg kg-1 b.w.; Metformin at 100 mg kg-1 b.w. (positive control) and distilled water consumed group was negative control. Thirty minutes after oral treatment, 2 g kg-1 b.w. of glucose was given to the experimental animals. Then, the glucose level in blood of the groups at the time points 0, 30, 60 and 120 minutes was determined.
Statistical analysis: All data were expressed as mean ± S.E. Results were analyzed by one-way analysis of variance (ANOVA), and significant differences were determined by Student’s t- test. Differences were statistically significant at P<0.05.
III. RESULTS AND DISCUSSION
α-glucosidase and α-amylase inhibition
Table 1.
The effect of VAM on α-glucosidase and α-amylase inhibitory activities
Sample |
Concentration (μg mL-1) |
% Inhibition of α-glucosidase |
% Inhibition of α-amylase |
VAM |
100 |
6.4 ± 0.82 |
- |
500 |
24.55 ± 0.6 |
15.25 ± 0.91 |
|
Acarbose* |
100 |
9.69 ± 2.28 |
71.09 ± 0.42 |
500 |
45.18 ± 0.67 |
98.06 ± 0.21 |
The results in Table 1 showed that VAM had a weak effect on inhibiting α-glucosidase enzyme at concentration of 500 μg mL-1. Acarbose was used as the positive control that was a significant α-glucosidase inhibitor at concentration of 100 μg mL-1 and 500 μg mL-1. In addition, α-amylase inhibition studies demonstrated that VAM had had nearly no effect at concentrations of 100 mg mL-1 and 500 mg mL-1 (Table 2). In all experiments, control samples were prepared accordingly without VAM and were compared with the tested concentrations of VAM. For acarbose treatment at concentrations of 100 μg mL-1 and 500 μg mL-1, α-amylase enzyme was inhibited considerably (up to 98%).
VAM contained six main compounds of the class C29-steroid and their structure was established based on different spectra and after comparing obtained spectra with spectroscopic data. Vernoniacums B, vernoniacums B1 and vernoniacums B2 were reported in the previous studies [6]. Vernomyoside C and vernomyoside C1 was known as the synthesized compounds in turn from vernoniacums B2 and vernoniacums B1 and firstly isolated from V. amygdalina in nature, which strongly inhibited α-amylase enzyme at concentration of 200 μg mL-1 with inhibition values of 82.02% and 78.85%, respectively. Besides, a new compound from V. amygdalina described as vernoamyoside E that significantly supressed both α-amylase and α-glucosidase enzymes [6,14]. Vernoamyoside E inhibited 50.99% of α-amylase enzyme at concentration of 200 μg/mL and decreased α-glucosidase enzyme at concentration of 500 μg mL-1 by 60.03%.
In the previous study, the ethanol extract of V. amygdalina leaf grown in Malaysia was reported that had effect on inhibiting both α-amylase and α-glucosidase enzymes with IC50 values of 510 μg mL-1 and 490 μg mL-1, respectively [15]. According to Hassan and colleague (2020), the methanol extract of V. amygdalina leaf from Keffi, Nigeria was a effective inhibitor of α-amylase enzyme by reducing 70.54% [16]. Besides, in Benin City, Nigeria, methanol extract of V. amygdalina root was also demonstrated as an inhibitor of α-amylase and α-glucosidase enzymes with IC50 values of 18.17 μg mL-1 and 12.17 μg mL-1, respectively [17].
Acute toxicity
There was no mortality as well as the abnormal clinical signals observed in control and VAM treated groups. The symptoms such as irregular movement, fatigue, and coma did not express in all tested mice. During 7 days of experiment, mice treated with VAM have consumed food and water similar to the biological control group. As shown in Table 1, the weight of mice in all investigated groups was not different at each recorded time points. The obtrained results showed that VAM did not cause any acute toxic effects to treated mice, even at the highest oral dose as 10 g kg-1 b.w.
Table 2.
The effect of VAM on body weight of mice in acute toxicity test
Experimental Groups |
Mean of body weight (gram) ± SE |
|||
Day 0 |
Day 1 |
Day 4 |
Day 7 |
|
Biological Control |
20.31 ± 0.28 |
20.17 ± 0.32 |
20.89 ± 0.40 |
22.03 ± 0.35 |
VAM (5 g kg-1) |
20.43 ± 0.31 |
20.23 ± 0.37 |
20.92 ± 0.31 |
22.22 ± 0.43 |
VAM (6 g kg-1) |
20.78 ± 0.33 |
20.59 ± 0.42 |
21.33 ± 0.36 |
22.26 ± 0.40 |
VAM (7 g kg-1) |
20.45 ± 0.51 |
20.23 ± 0.36 |
21.02 ± 0.43 |
22.08 ± 0.41 |
VAM (8 g kg-1) |
20.37 ± 0.38 |
20.15 ± 0.30 |
20.96 ± 0.44 |
22.09 ± 0.43 |
VAM (9 g kg-1) |
20.66 ± 0.42 |
20.31 ± 0.32 |
21.13 ± 0.34 |
22.15 ± 0.38 |
VAM (10 g kg-1) |
20.47 ± 0.31 |
20.11 ± 0.44 |
21.08 ± 0.40 |
22.12 ± 0.45 |
P |
>0.05 |
>0.05 |
>0.05 |
>0.05 |
Hyperglycemic reductive activity
From the beginning to 72 hours after alloxan monohydrate injection, the average weight of mice in the tested groups did not differ from the control group (P>0.05). After 7 days of induced hyperglycemia, the experimental mice in the control group, metformin treated group (100 mg kg-1 b.w day-1) and the VAM consumed groups (dose of 100, 200 and 400 mg kg-1 b.w day-1) were not significantly different (P> 0.05).
Table 3.
Body weight and blood glucose level of experimental hyperglycemic mice after treatment with VAM
Experiments |
Mean body weight (Gram) ± SE |
Blood glucose levels (mmol L-1) ± SE |
|||
Before Alloxan injection |
After 3 days Alloxan injection |
After 7 days VAM treatment |
Before VAM treatment |
After 7 days of VAM treatment |
|
Negative control |
28.66 ± 1.09 |
26.08 ± 1.08 |
26.29 ± 1.48 |
16.60 ± 3.70 |
16.50 ± 1.29 |
Reference control |
28.40 ± 1.24 |
27.08 ± 1.71 |
26.45 ± 2.09 |
16.34 ± 3.35 |
11.40*± 0.99 |
VAM 100 mg kg-1day-1 |
28.94 ± 2.03 |
27.66 ± 1.51 |
28.22 ± 2.33 |
16.38 ± 4.48 |
13.64 ± 1.83 |
VAM 200 mg kg-1day-1 |
28.62 ± 1.67 |
26.56 ± 1.91 |
27.22 ± 2.11 |
16.60 ± 4.61 |
13.80 ± 1.96 |
VAM 400 mg kg-1day-1 |
28.60 ± 0.73 |
26.82 ± 2.32 |
26.46 ± 2.59 |
16.56 ± 3.91 |
12.82* ± 1.10 |
P |
>0.05 |
>0.05 |
>0.05 |
*P <0.05 |
As shown in Table 2, the blood glucose levels in VAM and metformin treated groups had reduced significantly compared to that of untreated control group. Metformin at the dose of 100 mg kg-1day-1 decreased the blood glucose level of experimental mice significantly from 16.34 ± 3.35 mmol L-1 to 11.40* ± 0.99 mmol L-1 (P<0.05). Besides, the VAM treated mice at the dose of 100 mg kg-1day-1 and 200 mg kg-1day-1 presented the reduction of the glucose level from (16.38 ± 4.48 mmol L-1) and (16.60± 4.61 mmol L-1) to (13.64 ± 1.83 mmol L-1) and (13.80 ± 1.96 mmol L-1) respectively. However, this decrement was insignificant in comparison with the negative group (P>0.05). Nevertheless, VAM at the concentration of 400 mg kg-1day- induced the significant decrease of blood glucose level after 7-days treatment from 16.56 ± 3.91 mmol L-1 to 12.82 ± 1.10 mmol L-1 (P<0.05).
Anti-glucose tolerance activity
Based on the above anti-hyperglycemic results, VAM at the dose of 400 mg kg-1 b.w. were subjected to the anti-glucose tolerance test. As reported in the Table 3, VAM treated mice had not expressed the ability of resistance to glucose tolerance in compared with pathological control at all tested time points. The blood glucose level of mice treated with metformin (positive control group) was reduced significantly at all experimented checking points.
Table 4.
The concentration of blood glucose in mice at several time points
Experimental Groups |
Blood glucose levels (mg/dL) ± SE |
|||
T0 |
T30 |
T60 |
T120 |
|
Negative Control |
72.72 ± 1.94 |
263.16 ± 29.35 |
275.76 ± 28.80 |
206.55 ± 15.14 |
Metformin (100 mg kg-1) |
72.72 ± 2.58 |
181.08 ± 3.49* |
174.96 ± 3.96* |
138.96 ± 4.91* |
VAM (400 mg kg-1) |
73.44 ± 2.98 |
328.05 ±14.31 |
290.52 ± 31.51 |
201.0 ± 8.99 |
* means P <0.05 comparing with pathological control
Serum-lipid lowering
Results from Table 4 exhibited that VAM at 400 mg kg-1 could significantly reduced total cholesterol and LDL-cholesterol levels. VAM at this concentration also exhibited decreasing activity of triglyceride effectively and even reduced HDL-cholesterol level. Atorvastatin as positive control played a significant function in lowering cholesterol, triglyceride and LDL-cholesterol parameters.
Table 5.
Level of lipid in blood after 24 hours of treatment
Experimental Groups |
Cholesterol (mmol L-1) |
Triglyceride (mmol L-1) |
HDL- Cholesterol (mmol L-1) |
LDL- Cholesterol (mmol L-1) |
Physiological Control |
2.60 ± 0.09 |
1.30 ± 0.08 |
1.60 ± 0.07 |
1.00 ± 0.06 |
Pathological Control |
15.60 ± 0.51 |
18.70 ± 0.89 |
5.40 ± 0.35 |
1.60 ± 0.07 |
Atorvastatin (50 mg kg-1) |
9.21* ± 0.31 (↓40,96) |
10.68* ± 0.34 (↓42.89) |
5.40 ± 0.19 |
1.10* ± 0.06 (↓31.25%) |
VAM (400 mg kg-1) |
9.50* ± 0.69 (↓39.10%) |
18.65 ± 0.86 (↓0.27%) |
3.30 ± 0.27 (↓33.89%) |
1.30* ± 0.11 (↓18.75%) |
*P <0.05 compared with the pathological control
According to GSH (Globally Harmonized System of Classification and Labelling of Chemicals, 2011), the methanol extract from V. amygdalina Del. (VAM) has not been determined concentration leading to death in a half of tested animals or LD50 higher than 5000 mg kg-1, that was considered as relative low acute toxicity or unclassified. Based on this results, the selected safe doses for further pharmaceutical studies in animal models were 100 - 200 - 400 mg kg-1. In this study, VAM were determined its effects on anti-hyperglycemic as well as hyperlipidemic reductive activities. The groups of mice treated with VAM at all tested concentrations, which were 100 - 200 - 400 mg kg-1, showed a down regulation glucose levels in alloxan induced hyperglycemic mice. Moreover, VAM at the oral dose of 400 mg kg-1 could either reduce blood glucose level or LDL-cholesterol in poloxamer P407 induced hyperlipidemic mice. As widely recognized, glucose and low-density lipoprotein were seemly a major factor in artherogenesis and a main cause of cardiovascular disease among cases with diabetes [18]. By supplementing 15% the powder of V. amygdalina Del. leaves to daily diet, Atangwho et al., (2012) demonstrated that V. amygdalina Del. significantly reduced total cholesterol and glucose in serum of fattened animals with high fat diet model [19]. Beside, chloroform extract of this plant played function in significantly suppressing some major enzymes of diabetic disease in rats treated with 200 and 400 mg for 14 days [20]. Other scientists carried out investigation of antidiabetic effect of V. amygdalina in vivo. The antidiabetic effects between ethanolic leaf extracts of old and young V. amygdalina on streptozotocin (STZ) induced diabetic rat for four weeks were evaluated. Results from treated rats revealed that the higher dose (300 mg kg-1) of both extracts significantly (P<0.05) reduced serum ALT, AST, and ALP levels, and significant (P<0.05) decrease in LDL-C and VLDL-C in the extract-treated rats with a corresponding increase in HDL-C, as compared to the diabetic control rats [21]. The V. amygdalina leaf aqueous extract expressed hypoglycemic effect by significantly reducing the blood glucose level in diabetic treated rats. Oral intervention of V. amygdalina leaf aqueous extract averts dyslipidemia, and amplified serum HDL-cholesterol level, has the effect of preventing hepatocellular injury and obstructive liver disease in liver damaged conditions in a diabetic state [22]. Methanolic extract of leaf V. amygdalina growing in Vietnam showed α-glucosidase and α-amylase inhibition activity as well as cytotoxic activity against two cancer cell lines Hep3B and MCF-7 with IC50 value was 114.82 ± 2.13 µg mL-1 and 123.03 ± 1.27 µg mL-1, respectively (Hien et al, 2018). A new steroid saponin named vernoamyoside E was isolated from the leaves of the Vietnamese V. amygdalina and significantly inhibited both against a-amylase and a-glucosidase activity [6].
In this study, the leaf methanolic extract of V. amygdalina Del. at concentration 100 and at 400 mg kg-1 significantly reduced blood glucose level of induced hyperglycemic mice. This was first report of anti-glucose tolerance activity as well as serum-lipid lowering capacity of leaf methanolic extract of V. amygdalina Del. growing in Vietnam, by significantly decrease of blood glucose level and LDL-cholesterol, respectively.
IV. CONCLUSIONS
The obtained results in this report and from others of our works demonstrate that Vietnamese V. amygdalina possesses different potentials which were very useful for pharmaceutical purposes. The methanol leaf extract of this plant has great potential for using in supplementary treatment to oral hypoglycemic and antihyperlipidemic drugs for management of diabetes patients. Further studies are needed to find substances with specific activity in the treatment of common diseases such as diabetes mellitus clinically.
References:
- Chaudhury A, Duvoor C, Reddy Dendi VS, et al. (2017) Clinical Review of Antidiabetic Drugs: Implications for Type 2 Diabetes Mellitus Management. Front Endocrinol (8): 6.
- Glenn NL, ed. (2014) Cardiology Secrets. Texas, US: Saunders: 306-311.
- Graham RB (2014) Lipids and disorders of lipoprotein metabolism. In: William JM, Andrew PD, Marta L, Ruth MA, eds. Clinical Biochemistry: Metabolic and Clinical Aspects. 3rd ed. London, UK: Churchill Livingstone: 702-736
- Campese, V. M., & Raju, S. (2012). Altering Serum Lipids to Reduce Progression of Chronic Kidney Disease. Nutritional Management of Renal Disease, 241.
- Hien NK, Tran HPD, Anh HLT, et al. (2018) The plant Vernonia amigdaina D and its biochemicals. Vietnam Trade Ind Rev. (14): 412-417.
- Anh HLT, Vinh LB, Lien LT, et al (2019) In vitro study on α-amylase inhibitory and α-glucosidase of a new stigmastane-type steroid saponin from the leaves of Vernonia amygdalina. Nat Prod Res.: 1-7.
- Tundis R, Loizzo MR, Menichini F (2010) Natural products as α-amylase and α-glucosidase inhibitors and their hypoglycaemic potential in the treatment of diabetes: an update. Mini Rev Med Chem 10(4): 315-331.
- Elya, B., Handayani, R., Sauriasari, R., Hasyyati, U. S., Permana, I. T., & Permatasari, Y. I. (2015). Antidiabetic activity and phytochemical screening of extracts from Indonesian plants by inhibition of alpha amylase, alpha glucosidase and dipeptidyl peptidase IV. Pakistan Journal of Biological Sciences, 18(6), 279.
- Paduchuri PP, Duvvuri VL, George P (1975) Acute Toxicity Studies of a newly developed anti-obesity polyherbal formulation in Zebrafish. Int. J. Pharm Biol. Sci. 9(1): 133-136.
- Mahdy A and Shehab NG (2015) Hypoglycemic Activity of Fagonia indica and Aloe vera in Alloxan-Induced Hyperglycemia in Mice. EC. J. Pharm Sci. 2: 239-244.
- Sivalingam G, Sriram N (2013) Anti-diabetic activity of Ribes nigrum fruit extract in alloxan induced diabetic rats. Int. J. Pharm Sci. 4(3): 1196-1201.
- Palmer WK, Emeson EE, Johnston TP (1998). Poloxamer 407-induced atherogenesis in the C57BL/6 mouse. Atherosclerosis 136(1): 115-123.
- Sheneni VD, Odiba VA, Omede A, et al. (2018) Anti-hyperlipidemic effect of Vitex doniana in poloxamer induced hyperlipidemia. MOJ. Biol. Med 3(4): 168-173.
- Anh, H. L. T. (2018). Sterols and flavone from the leaves of Vernonia amygdalina del. growing in thua thien hue. Vietnam Journal of Science and Technology, 56(6), 681.
- Alara OR and Abudurahman NH (2019) Anti-diabetic activity and mineral elements evaluation of Vernonia amygdalina leaves obtained from Malaysia. J Res Pharm, 23(3)P: 514-521.
- Hassan, M. (2020) Evaluation of alpha amylase inhibitory activity of the ethanol extract of some locally sourced plants wth known hypoglycaemic properties. Journal of Biologics Research and Development Vol 1(1).
- Igbinidu, G. O. (2019). GC-MS analysis, phytochemical screening and In vitro alpha amylase and alpha glucosidase inhibitory activities of Vernonia amygdalina root extract and fractions. Journal of Pharmacognosy and Phytochemistry, 8(4), 2125-2131.
- Wang Y, Lammi-Keefe CJ, Hou L, et al. (2013) Impact of low-density lipoprotein cholesterol on cardiovascular outcomes in people with type 2 diabetes: a meta-analysis of prospective cohort studies. Diabetes Res Clin Pract. 102(1): 65-75.
- Atangwho IJ, Edet EE, Uti DE, et al. (2012) Biochemical and histological impact of Vernonia amygdalina supplemented diet in obese rats. Saudi J Biol Sci. 19(3): 385-392.
- Atangwho, I. J., Yin, K. B., Umar, M. I., Ahmad, M., & Asmawi, M. Z. (2014). Vernonia amygdalina simultaneously suppresses gluconeogenesis and potentiates glucose oxidation via the pentose phosphate pathway in streptozotocin-induced diabetic rats. BMC complementary and alternative medicine, 14(1), 1-13.
- Asante DB, Effah-Yeboah E, Barnes P, et al. (2016) Antidiabetic effect of young and old ethanolic leaf extracts of Vernonia amygdalina: A comparative study. J Diabetes Res.: 1-13.
- Akawa AB, Gazuwa SY, Ojo AB et al. (2018) Effects of aqueous extract of Vernonia amygdalina Delile leaves in Alloxan induced diabetic rats. Pharmacology Online 2: 219-226.