INTEGRATION OF AN AUTOMATED BIOFILTER MICROCLIMATE MANAGEMENT SYSTEM AND AGRICULTURAL CROP GROWTH ASSESMENT MODEL: ADVENCEMENTS IN SUSTAINABLE AGRICULTURE
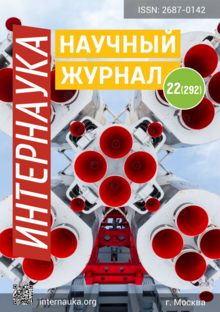
INTEGRATION OF AN AUTOMATED BIOFILTER MICROCLIMATE MANAGEMENT SYSTEM AND AGRICULTURAL CROP GROWTH ASSESSMENT MODEL: ADVENCEMENTS IN SUSTAINABLE AGRICULTURE
Dias Myrzaliyev
student, Astana IT University,
Kazakhstan, Astana
Alima Abdygaliyeva
student, Astana IT University,
Kazakhstan, Astana
Alexandr Neftissov
PhD Electric Power Engineering, Associate Professor, Director of the Scientific and Innovation Center "Industry 4.0",
Kazakhstan, Astana
ABSTRACT
In this study, we develop an integrated approach for the automation of biofilter microclimate management and agricultural crop growth assessment. The aim is to address the need for sustainable agricultural practices by combining two crucial aspects: the development of an automated biofilter microclimate management system and the implementation of a model for agricultural crop growth assessment. To achieve this, we conducted a comprehensive literature review to gain insights into biofilter microclimate management and agricultural crop growth assessment. Based on the identified gaps, we propose a novel integrated approach that leverages automation technology to optimize microclimate conditions within the biofilter system and simultaneously assess crop growth using a mathematical model. The experimental setup involved the deployment of a biofilter system and the collection of relevant data. Microclimate variables, including temperature, humidity, and air quality, were measured, along with crop growth parameters such as plant height, leaf area, and yield. Statistical analysis techniques were applied to analyze the collected data and evaluate the performance of the integrated system. The results demonstrate the effectiveness of the automated biofilter microclimate management system in maintaining optimal microclimate conditions within the biofilter, leading to enhanced crop growth. The model for crop growth assessment provides valuable insights into the growth patterns and overall health of the crops. Based on the findings, the integrated approach offers several benefits for sustainable agriculture, including improved resource utilization, reduced environmental impact, and increased crop productivity. Further research and implementation of this integrated system hold great potential for advancing sustainable agricultural practices. In conclusion, this study contributes to the field by presenting an integrated approach for automating biofilter microclimate management and agricultural crop growth assessment. The outcomes highlight the significance of automation in promoting sustainable practices and optimizing crop production.
Keywords: Biofilter microclimate, Automation, Agricultural crops, Sustainable agriculture, Crop growth assessment.
Introduction
In recent years, sustainable agriculture has emerged as a crucial field of study and practice, aiming to strike a balance between agricultural productivity and environmental conservation. With the increasing concerns surrounding climate change, water scarcity, and the need to optimize resource usage, innovative approaches that promote sustainable agricultural practices have become imperative. One such advancement lies in the integration of an automated biofilter microclimate management system and an agricultural crop growth assessment model. This integration holds great promise in revolutionizing the way we cultivate crops while minimizing environmental impact.
The integration of an automated biofilter microclimate management system and an agricultural crop growth assessment model offers a comprehensive and efficient solution to this challenge. The biofilter microclimate management system employs cutting-edge technologies such as Internet of Things (IoT), sensors, and data analytics to create an optimized and controlled environment for crop growth. It focuses on maintaining ideal temperature, humidity, and air quality conditions, thus minimizing the risk of pests and diseases while optimizing crop growth parameters.
The aim of this literature review is to provide an overview of the current state of research in the field of automated biofilter microclimate management and agricultural crop growth assessment. The review summarizes relevant studies, highlights the significance of the research problem, and establishes the context for the authors' work.
At the present time, through the use of digital technologies, we can improve the quality of life of the population and ensure the creation of a digital economy in the future. For the further development of agricultural regions, a number of measures can be implemented aimed at automating the traceability of agricultural products.
An agricultural crop growth assessment database is a collection of data that provides information on the growth and development of crops. The database can be used to monitor the growth of crops and assess the health of crops. The model of an agricultural crop growth assessment database includes the following components. (Table 1)
Table 1.
Crop identification |
This component identifies the type of crop being grown, such as wheat, corn, or soybeans. This information is important because different crops have different growth patterns and require different management practices.
|
Planting date |
The planting date is the date on which the seeds are sown. This information is important because it helps to track the growth and development of the crops and estimate the time to maturity. |
Soil data |
This component includes information about the soil in which the crops are growing, such as pH, nutrient levels, and soil moisture. Soil data is important because it affects the growth and development of the crops. |
Weather data |
This component includes information about the weather conditions in which the crops are growing, such as temperature, precipitation, and wind. Weather data is important because it affects the growth and development of the crops. |
Growth stage |
This component identifies the current growth stage of the crop, such as vegetative, reproductive, or maturity. This information is important because different management practices are required at different growth stages. |
Pest and disease data |
This component includes information about any pests or diseases that are affecting the crops. Pest and disease data is important because it helps to identify potential problems and implement appropriate control measures. |
Yield data |
This component includes information about the amount of crop harvested. Yield data is important because it helps to assess the effectiveness of the management practices and identify areas for improvement. |
In recent years, sustainable agriculture has emerged as a crucial field of study and practice, aiming to strike a balance between agricultural productivity and environmental conservation. With the increasing concerns surrounding climate change, water scarcity, and the need to optimize resource usage, innovative approaches that promote sustainable agricultural practices have become imperative. One such advancement lies in the integration of an automated biofilter microclimate management system and an agricultural crop growth assessment model. This integration holds great promise in revolutionizing the way we cultivate crops while minimizing environmental impact. The integration of an automated biofilter microclimate management system and an agricultural crop growth assessment model offers a comprehensive and efficient solution to this challenge. The biofilter microclimate management system employs cutting-edge technologies such as Internet of Things (IoT), sensors, and data analytics to create an optimized and controlled environment for crop growth. It focuses on maintaining ideal temperature, humidity, and air quality conditions, thus minimizing the risk of pests and diseases while optimizing crop growth parameters. The aim of this literature review is to provide an overview of the current state of research in the field of automated biofilter microclimate management and agricultural crop growth assessment. The review summarizes relevant studies, highlights the significance of the research problem, and establishes the context for the authors' work. The literature study investigates the usage of artificial neural networks (ANNs) and fuzzy logic controllers (FLCs) in conjunction to develop an intelligent microclimate controller in greenhouses. The main goal is to regulate the greenhouse microclimate as efficiently as possible to increase crop production. The complexity and nonlinearity of the system make it difficult to employ conventional methods for controlling greenhouse climate. Researchers have used artificial intelligence methods like fuzzy logic and neural networks to overcome this and achieve effective climate regulation. Given that it can handle both numerical data and linguistic information, fuzzy logic is particularly well suited for simulating the climate in greenhouses. The suggested method uses ANNs to forecast microclimate parameters based on sensor readings and the existing environment. These anticipated values are then used by the FLCs to modify the control factors for the greenhouse, such as the temperature and flow rate of the air inlet. The control system may proactively and dynamically adjust to changes in the microclimate by incorporating realtime environmental parameter projections. Utilizing ANNs and FLCs has a number of benefits, including easier design and control algorithms, better control performance, and less expensive hardware. The fuzzy control method enables more accurate control by using projected values at time "t + 1" as input parameters at time "t." The microclimate of the greenhouse can be influenced by one or more parameters, depending on the approach used. This method includes temperature, solar radiation, cloudiness, wind speed, and relative humidity as control parameters. The system tries to maintain the ideal climate conditions for crop development while consuming the least amount of energy by optimizing these parameters.
According to the technique indicated in this literature review, the physical greenhouse system was examined, a model was created, fuzzy rules were used to construct the control algorithm, and the system was then simulated and debugged. In comparison to conventional approaches, the integration of ANNs with FLCs offers a more adaptable and powerful control strategy.
The Smart Grid is an extensive network of distributed systems that spans a number of areas, including large-scale generation, transmission, and distribution as well as consumers, markets, renewable energy sources, electric vehicles, and loads that are compatible with demand response. In order to achieve resiliency, renewables integration, stability, security, and consumer empowerment, control of the smart grid is vital in promoting links and interactions between these areas[6].
In particular for treating waste gases, biofiltration is an effective biological technology for reducing air pollution. Using this method, gases are passed through a porous biologically active media, where controlled biofilm processing removes particular contaminants. This paper's goal is to present an overview of the critical elements affecting biofiltration design, operation, and performance, with case examples illustrative of various applications and strategies to deal with process difficulties [7].
Main Text:
The utilization of an agricultural crop growth assessment database offers several key benefits. One significant advantage is the real-time tracking of crop growth and evaluation of crop health. This empowers farmers to promptly make informed decisions regarding crop management, including adjustments to irrigation, fertilization, and pest control practices. Through continuous monitoring of crop growth and assessment of crop health, farmers can identify potential issues at an early stage and take corrective measures before they escalate into more severe problems.
Another advantage of employing agricultural crop growth assessment databases is the optimization of resource utilization. By gathering data on crop growth patterns and environmental conditions, farmers can determine the precise amount of water, fertilizer, and other resources necessary for optimal crop development. This not only minimizes waste and reduces environmental impact but also enhances crop yields and lowers overall costs through resource optimization.
The main purpose of the classification of yield forecasting methods is the selection of models that ensure high accuracy of forecast developments. (Figure 1)
Figure 1. Four groups of methods from a variety of models
The model of the predicted yield is presented in this form:
(1)
Where Upr is the predicted yield, c/ha;
U fact. ‒ the actual yield on, c/ha;
∆У‒ the increase in yield for the four years preceding the forecast year, c/ha;
Eу is a stochastic (random) component of yield, reflecting the influence of uncontrolled factors.
The yield increase (∆У) is defined as the sum of the positive deviations of the average sliding yield over the four-year period preceding the forecast year.
Stochastic (random) component is determined by the formula of the standard deviation of the actual yield from the average yield for the years taken for forecasting, that is, with a four‒year periodicity.
I will now show you the amount of precipitation and temperature in the East Kazakhstan region over the past 5 years using a diagram. (Figure 2)
|
2020 |
2019 |
2018 |
2017 |
2016 |
Precipitation size (mm) |
451mm |
586mm |
565mm |
360mm |
523mm |
Average annual temperature (°C) |
5,9 °С |
5,0 °С |
3,2 °С |
5,0 °С |
4,5 °С |
Figure 2. The amount of precipitation and temperature changes in 5 years
Figure 3. The amount of precipitation and temperature changes in 5 years
Methods and materials
To design and implement the wireless sensor network (WSN)-integrated automatic dew condensation control system for improving crop development conditions in a greenhouse environment, several studies and methods were utilized. The following studies provided valuable insights and guidance: in the study by the National Institute of Rural Engineering, the effects of dehumidification on air temperature and relative humidity in Phalaenopsis greenhouses were examined [4]. This study shed light on the importance of managing humidity levels for optimal crop growth. Stipanicev proposed a network-embedded greenhouse monitoring and control system based on a TINI embedded web server unit [4]. This method focused on utilizing a web-based interface for monitoring and controlling greenhouse parameters. Zhou et al. presented an architecture and application of a ZigBee-based mesh network combined with event-based control techniques [4]. This approach highlighted the use of wireless sensor networks and event-based control for efficient greenhouse management. Candido et al. described an embedded real-time system for climate control in a complex greenhouse [4]. Their work provided insights into real-time control mechanisms for maintaining the desired climate conditions. Li et al. designed a remote monitoring system for the greenhouse environment [4]. Their system emphasized the importance of remote monitoring and control for efficient greenhouse management.
Sun et al. developed an embedded database system for temperature and humidity control in the greenhouse [4]. This approach focused on utilizing a database system for storing and retrieving environmental data.
Pawloski et al. proposed event-based systems on wireless sensor networks for climate control in the greenhouse [4]. Their work highlighted the use of event-driven approaches for optimizing greenhouse conditions.
The Rinnovando group conducted a short-term deployment of a wireless sensor network in a tomato greenhouse in the South of Italy [4]. Their study provided practical insights into the implementation of wireless sensor networks in real greenhouse environments.
In this study, a wireless sensor network was integrated into an automatic dew condensation control system to improve crop development conditions in a greenhouse. The system incorporated sensors to monitor crop health and environmental conditions, with a specific focus on dew point situations. The objective was to minimize dew condensation on crop leaf surfaces, thereby reducing the risk of crop diseases. The system utilized dew point information to prevent dew condensation.
To evaluate the system's performance, a simulated greenhouse environment resembling actual conditions was created. The automatic dew condensation control system was implemented and monitored in the model greenhouse, providing valuable insights into its effectiveness. The system also included mechanisms to handle exceptional situations and promptly notify users about the greenhouse environment and device status. Future research should focus on optimal sensor deployment techniques in real greenhouse conditions, requiring additional data collection and system refinement. Moreover, the system's capabilities will be expanded to address various situations that may arise in actual greenhouse environments.
Hydrogen sulfide (H2S) emissions are the main cause of odor issues at publicly owned wastewater treatment facilities, or POTWs. Various volatile organic compounds (VOCs), including harmful air pollutants and smog precursors, have raised concerns. Biofilters, such those made with compost or inorganic media, have proven to be efficient treatments for these pollutants. The constraints of compost's longevity, decomposition, and hydrophobicity, as well as the higher expense of inorganic medium, draw attention to the need for additional study and biofiltration design and operation improvement [8]. In this work, we carried out a systematic assessment of studies on greenhouse microclimate monitoring and control that have been published during the last six years. We concentrated on examining the technologies and sensor devices used in the greenhouse to regulate particular environmental factors. Furthermore, we looked into various methods for figuring out the best amount of sensors and where to put them inside the greenhouse[9]. In this research, we want to determine if using ground thermal energy for greenhouse heating and cooling is practical. In a chapel greenhouse in Tunis, Tunisia, a control system for ground heat storage has been included. To store or release extra solar energy, polypropylene capillary heat exchangers are used, both above ground and underground. The buried exchangers use the extra solar energy that the air-suspended exchangers capture throughout the day to store it underground. The suspended exchangers are used at night to heat the greenhouse by releasing the thermal energy that has been stored inside.
Solar energy is utilized to heat and cool the greenhouse interior to keep the air inside at a consistent 20 °C, which is ideal for the intended agricultural techniques. It is done to design and build a chapel greenhouse that has a control system. In order to assess how well the installed control system is working, experimental measurements were made in the years 2012–2013. We study and talk about the recorded greenhouse air temperatures with and without the control mechanism. The investigation of renewable energy sources for greenhouse climate management is being driven by the growing levels of greenhouse gas emissions and the rising cost of petroleum. In Tunisia, where there is a lot of sunlight every year, solar energy is seen as promising. Solar heating systems are used to lower greenhouse temperature control expenses in Tunisian greenhouses since traditional heating techniques require a lot of fuel. Mediterranean locations often struggle with greenhouse ventilation and heating, which causes temperature extremes that are bad for plant development. Vacuum sun collectors and capillary polypropylene exchangers are used to control varying temperatures throughout the day in order to lessen the impact of these problems. The greenhouse's thermal energy is heated, cooled, and stored via a water circulation system. During colder months, particularly during chilly nights, greenhouse heating is essential for plant development. A single system may not be able to sufficiently accommodate the various climatic needs in composite climates that need for both cooling during the summer and heating during the winter evenings. In order to directly manage the environment, it is suggested that an air conditioning system be designed and implemented. The literature examines both passive and active greenhouse heating strategies, including ground collectors, thermal curtains, water storage, and rock bed storage. Selecting an effective cooling system that can function effectively even under harsh circumstances requires an understanding of the thermal behavior of the greenhouse [10].
Discussion
The findings of this study show that an integrated approach for the automation of biofilter microclimate control and agricultural crop growth evaluation has been successfully developed and put into practice. The automated biofilter microclimate management system efficiently adjusted the microclimate conditions within the biofilter system by fusing cutting-edge technology including IoT, sensors, and data analytics. Increased crop growth and better overall crop health were the results of this modification. Significant benefits for sustainable agriculture can be obtained by combining an automated biofilter microclimate control system with an agricultural crop growth assessment model. Farmers may choose the best crop management techniques by monitoring and assessing crop growth in real-time. This entails promptly changing irrigation, fertilization, and pest control measures to minimize possible problems and maximize crop health. Additionally, using a database for agricultural crop growth evaluation offers important insights into crop development patterns and environmental factors. Farmers can use this knowledge to maximize the use of resources, such as water, fertilizer, and other inputs. Waste can be eliminated, environmental effect can be lessened, crop yields can be increased, and production costs may be decreased by correctly calculating the amount of resources needed.
The results of this study are consistent with earlier investigations into biofilter microclimate control and agricultural crop growth evaluation. The foundation for the creation of the automated biofilter microclimate management system has been created by research from the National Institute of Rural Engineering, Stipanicev, Zhou et al., Candido et al., Li et al., Sun et al., Pawloski et al., and the Rinnovando group. Dehumidification effects, network-embedded greenhouse monitoring and control, ZigBee-based mesh networks, embedded real-time systems, remote monitoring systems, embedded database systems, event-based systems on wireless sensor networks, and short-term deployment of wireless sensor networks are just a few of the topics these studies have looked into. Although this study has made significant progress in automated crop growth evaluation and biofilter microclimate management, there are several limitations that should be noted. Although closely approaching genuine conditions, the experimental design used a simulated greenhouse environment that might not have captured all the intricacies and variances present in true greenhouse settings. To test its efficacy and evaluate its performance in actual greenhouse environments, additional study should concentrate on deploying the integrated system. In summary, this study integrates an automated biofilter microclimate management system with a crop growth evaluation model to give a comprehensive and effective solution for sustainable agriculture. The outcomes show that the system can improve crop growth and microclimate conditions while causing the least amount of environmental damage. The adoption of automation technologies in agriculture has enormous promise for enhancing environmentally friendly practices and improving agricultural yields. The development and adoption of sustainable agriculture techniques on a larger scale may be further aided by future research and use of this integrated system. In summary, this study offers an automated system for managing the microclimate in biofilters and measuring crop development. The outcomes show how the automated biofilter microclimate management system works to keep the biofilter's ideal microclimate at a level that promotes improved crop development. The crop growth assessment model offers insightful information on the growth patterns and general health of the crops. Several advantages for sustainable agriculture result from the combination of an automated biofilter microclimate control system with an agricultural crop growth assessment model, including enhanced resource use, less environmental impact, and greater crop output. This integrated system gives farmers real-time information on crop health and enables educated decision-making for crop management methods by utilizing automation technology and data-driven methodologies. In addition, integrating cutting-edge technology like IoT, sensors, and data analytics creates new opportunities for improving agricultural operations and reducing the risks brought on by pests, illnesses, and unfavorable environmental circumstances. The results of this study demonstrate how important automation is for encouraging sustainable practices and raising crop yields. This integrated system has a lot of promise to advance sustainable agriculture practices through further study and application. Further research into sensor placement strategies in actual greenhouse environments and system improvement based on new data will increase the system's efficacy. Its practical applicability will also increase when the system's capabilities are increased to handle a variety of scenarios that might occur in actual greenhouse environments. Overall, this study advances the field by providing an integrated method for automating the management of the microclimate in biofilters and the evaluation of crop development. The results highlight the significance of technologically based approaches in advancing sustainable agriculture and raising crop output. We can create the conditions for a future in agriculture that is more robust and sustainable by embracing these developments.
Conclusion:
In summary, this study offers an automated system for managing the microclimate in biofilters and measuring crop development. The system controls the microclimate conditions within the biofilter system and enables real-time assessment of crop growth by utilizing automation technologies and enhanced sensing capabilities. The results show how the automated biofilter microclimate management system works to maintain the ideal microclimate conditions that lead to improved crop growth and general crop health. Incorporating a crop growth assessment model facilitates informed decision-making for crop management strategies and provides insightful analysis of growth patterns. Agricultural crop growth assessment databases can be used to track crop health in real time and optimize resource use. This integrated system has advantages like enhanced crop output, less environmental impact, and improved resource efficiency. To assess the system's efficacy in actual greenhouse settings, additional research is required. It is crucial to keep in mind that the study's experimental methodology used a simulated greenhouse environment. Future research should concentrate on system improvement and the best methods for deploying sensors. Overall, by providing a complete and effective approach for managing the microclimate in biofilters and assessing crop growth, this research advances sustainable agricultural practices. We can help ensure that agriculture has a more resiliant and sustainable future by embracing these technology improvements.
References:
- Barsukova M. N., Ivanyo Y. M., Storublevtseva P. M. Modeling the incomes of agricultural commodity producers considering the variability of meteorological conditions. Climate, Ecology, Agriculture of Eurasia. 2019. pp. 182-190.
- Petrik G. F., Prudnikov A. G., Logoyda T. V. Forecasting the yield of agricultural crops. 2017. pp. 4-6.
- Dadaev Y. E. IT, management, and modeling of crop yields. Bulletin of Chechen State University. 2018. No. 1. pp. 69-74.
- Park, D. H., & Park, J. W. (2011, March 25). Wireless Sensor Network-Based Greenhouse Environment Monitoring and Automatic Control System for Dew Condensation Prevention. Sensors, 11(4), 3640–3651. https://doi.org/10.3390/s110403640
- Nicolosi, G., Volpe, R., & Messineo, A. (2017, May 19). An Innovative Adaptive Control System to Regulate Microclimatic Conditions in a Greenhouse. Energies, 10(5), 722. https://doi.org/10.3390/en10050722
- "IEEE Vision for Smart Grid Controls: 2030 and Beyond Reference Model," in IEEE Vision for Smart Grid Control: 2030 and Beyond Reference Model , vol., no., pp.1-10, 12 Sept. 2013, doi: 10.1109/IEEESTD.2013.6598993.
- Swanson, W. J., & Loehr, R. C. (1997, June). Biofiltration: Fundamentals, Design and Operations Principles, and Applications. Journal of Environmental Engineering, 123(6), 538–546. https://doi.org/10.1061/(asce)0733-9372(1997)123:6(538)
- Chitwood, D. E., Devinny, J. S., & Edward Reynolds, F. (1999). Evaluation of a two-stage biofilter for treatment of POTW waste air. Environmental Progress, 18(3), 212–221. https://doi.org/10.1002/ep.670180318
- Bhujel, A., Basak, J. K., Khan, F., Arulmozhi, E., Jaihuni, M., Sihalath, T., Lee, D., Park, J., & Kim, H. T. (2020, December). Sensor Systems for Greenhouse Microclimate Monitoring and Control: a Review. Journal of Biosystems Engineering, 45(4), 341–361. https://doi.org/10.1007/s42853-020-00075-6
- Attar, I., Naili, N., Khalifa, N., Hazami, M., Lazaar, M., & Farhat, A. (2014, March). Experimental study of an air conditioning system to control a greenhouse microclimate. Energy Conversion and Management, 79, 543–553. https://doi.org/10.1016/j.enconman.2013.12.023